By Alka Tripathy-Lang, Ph.D. (@DrAlkaTrip)
A remote Alaskan volcano erupted for nine months, repeatedly disrupting aviation traffic. How can lightning help minimize ash hazards for aircraft?
Citation Tripathy-Lang, Alka (2020), Volcanic lightning helps aviators avoid hazardous ash, Temblor, http://doi.org/10.32858/temblor.088
Plume from Bogoslof on February 19, 2017, seen from Unalaska Island, 53 miles east-southeast of the volcano. This photo, captured from a helicopter during fieldwork by the Alaska Volcano Observatory, was taken 14 minutes after this particular explosive event began. Credit: Janet Schaefer and the Alaska Volcano Observatory / Alaska Division of Geological & Geophysical Surveys
Pilot: KLM 867 heavy, we are descending now: we are in a fall!
On Dec. 14, 1989, Redoubt Volcano in Alaska began to erupt. The next day, in an effort to avoid ash clouds reported by other aircraft, KLM Flight 867 diverted from its normal route. At 11:46 a.m., the Boeing 747 inadvertently entered the ash cloud at approximately 25,000 feet. To exit the cloud, the crew decided to ascend, but after about 90 seconds, at 27,900 feet, all four engines failed. After gliding to 13,300 feet in a region where the mountains rise to as high as 11,000 feet, the crew managed to restart the engines and make an emergency landing in Anchorage, Alaska.
There were no casualties, but damage to the aircraft’s engines and electrical system from volcanic ash was more than $80 million. The National Transportation Safety Board investigated, and found, “A factor related to the accident was: the lack of available information about the ash cloud to all personnel involved.”
In recent years, scientists have developed and enhanced volcano monitoring tools, including those for stratospheric ash clouds. “Volcanic ash,” says Tom Gonzalez, a pilot for a US-based commercial airline, “is probably one of the worst things to deal with in aviation.”
Tracking volcanic eruptions
Alaska hosts over 50 historically active volcanoes according to the Alaska Volcano Observatory, all of which are capable of directly impacting air traffic should they erupt again. The Alaska Volcano Observatory monitors 30 of them in real time with seismic sensors placed directly on these volcanoes, but many potentially eruptive peaks lack direct monitoring because they are so remote. In these cases, scientists use additional tools to track activity, such as infrasound, satellite images, and more recently, volcanic lightning, according to Alexa Van Eaton, a volcanologist at the U.S. Geological Survey (USGS). These tools are used in concert to detect imminent or ongoing eruptions so that aviators can be alerted to any potential hazard.
One Alaskan volcano, and its recent prolonged eruption, highlights how volcanic lightning is giving scientists and aviators critical information to protect aircraft and passengers flying in regions prone to eruptions.
Bogosolf’s nine month long eruption
Though towering 19,500 feet (6 kilometers) above the seafloor, Bogoslof, a remote Alaskan volcano, pokes only 330-500 feet (100-150 meters) above the Bering Sea. After slumbering for more than 20 years, Bogoslof’s recent eruption began in December of 2016. This eruption lasted until August of 2017, and was punctuated by 70 explosions that produced ash plumes ranging from 13,000 to almost 39,000 feet (4 to almost 12 kilometers) above sea level, says Van Eaton.
During the months-long eruption of Bogoslof, 32 of the individual ash plume-generating explosions crackled with volcanic lightning that was detected almost instantaneously, worldwide, according to a paper lead by Van Eaton, published in the February issue of the Bulletin of Volcanology.
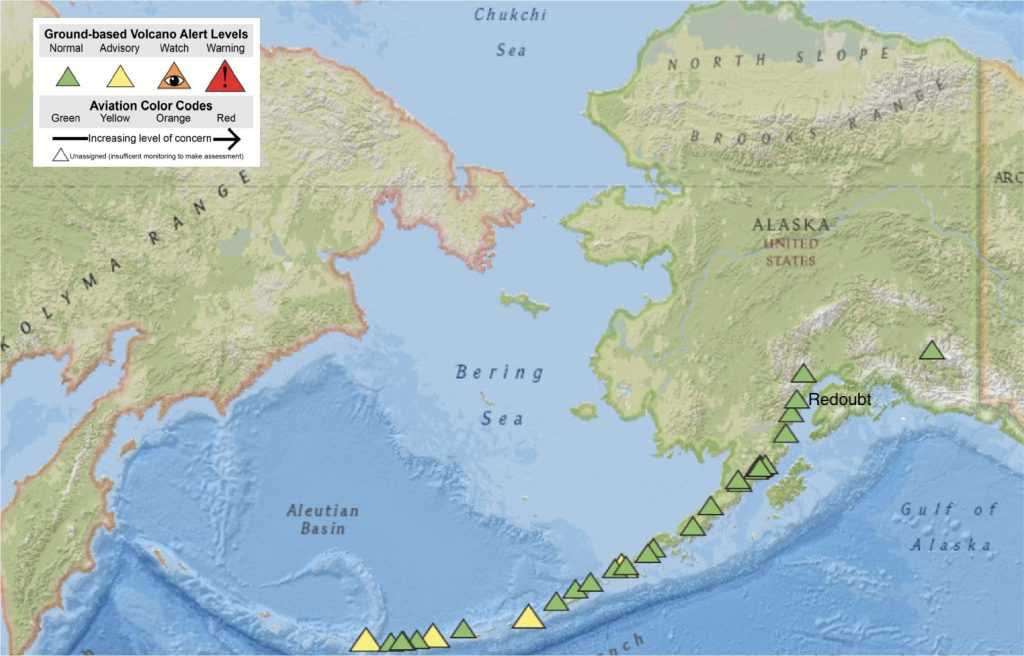
Detecting dirty thunderstorms
The mechanism by which volcanic lightning forms is much the same as thunderstorm lightning. Particles collide and are either stripped of their electrons or adorned with extras, becoming positively or negatively charged. As the newly charged particles separate, an electric field forms that is balanced by lightning.
For thunderstorms, ice is the necessary particle for ice-charging. In an eruption, as ash is catapulted from the vent into the tumultuous plume, silicate particles swap electrons in a process called silicate-charging. Volcanic lightning can form by either silicate charging only, or a combination of ice- and silicate-charging. The latter, which Van Eaton calls a “dirty thunderstorm,” happens when there’s an external source of water—like at Bogoslof. But how important is each mechanism?
Detection networks like the World Wide Lightning Location Network and Vaisala’s Global Lightning Dataset are designed to detect very low radio frequencies produced by lightning that propagate long distances at the speed of light, says Van Eaton. Between these two global networks, more than 4,550 volcanic lightning strokes were identified throughout Bogoslof’s eruption.
The plumes that hosted lightning shared two key characteristics—they rose above the atmospheric freezing level of water, which is —4 ºF (—20 ºC), and they were sustained for longer than 2 minutes. Higher summer temperatures drove water’s atmospheric freezing levels as high as 26,200 feet (8 kilometers), whereas in cold winter months, it dropped as low as 9,800 ft (3 kilometers). At Bogoslof, the occurrence of volcanic lightning depended strongly on altitude, implicating thunderstorm-style ice-charging as a major catalyst for Bogoslof’s lightning, says Van Eaton.
Commercial airliners typically fly at or above 30,000 feet, according to Gonzalez, who has flown numerous international flights while skirting actively erupting volcanoes. “Higher is usually better, but at times you may be in the low 30,000s due to winds, turbulence, or [another] aircraft condition.” At Bogoslof, all plumes higher than 29,500 feet (9 kilometers) produced lightning, regardless of season, indicating that when the plumes were high enough to interfere with aircraft, volcanic lightning tipped off scientists to the presence of ash.
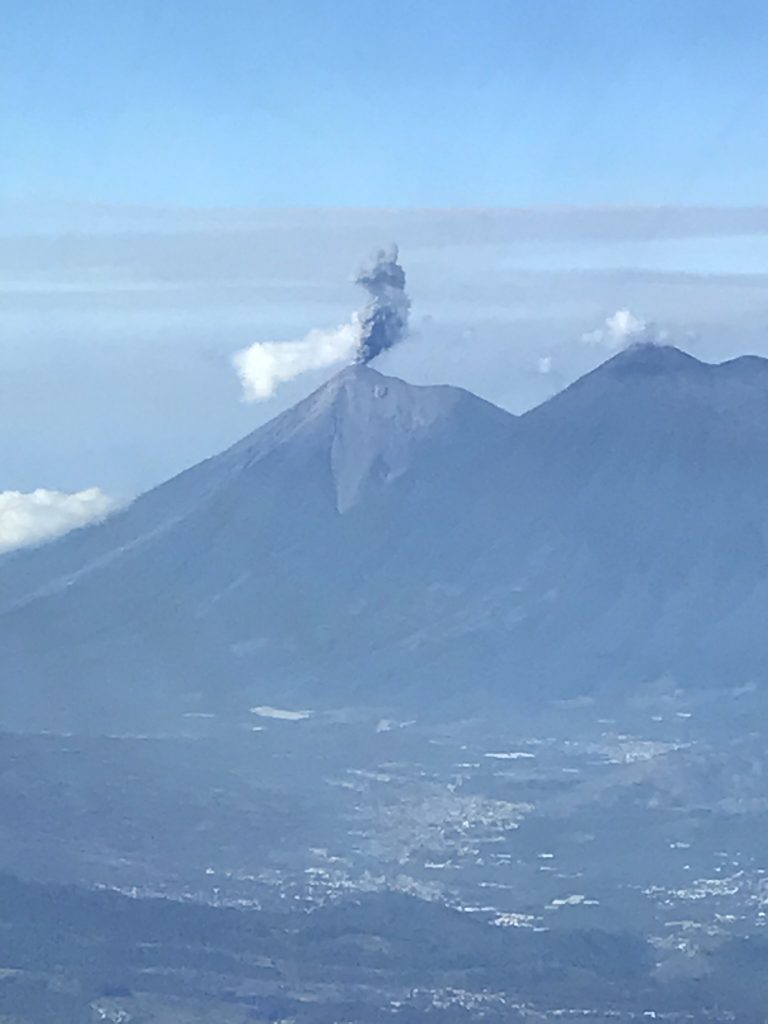
Combining techniques at Bogoslof
Dave Schneider, a research geophysicist at the Alaska Volcano Observatory, was first alerted to Bogoslof’s eruption as pilots flew by and notified the Federal Aviation Administration (FAA) dispatchers of peculiar activity. Once scientists knew Bogoslof was erupting, they applied their full arsenal of remote monitoring tools, including volcanic lightning detection, to evaluate the hazard to aircraft from individual explosions for the duration of the eruption.
Plume height and location – critical details for aviators – is most often deduced from satellite imagery. But in these images, Bogoslof’s plumes often lacked tell-tale ash signatures, giving the illusion that these were plumes of harmless water as ash rained on nearby settlements, as summarized in a parallel paper lead by Schneider. If an explosion at Bogoslof was accompanied by lightning, van Eaton says, “we know it was an ashy [explosion].”
“Time delays for new satellite images can be up to 15 to 30 minutes depending on the satellite, and if the weather is bad, it may be impossible to see the volcanic cloud anyway,” Van Eaton says. On the other hand, detection of volcanic lightning is nearly instantaneous, no matter the visibility conditions, and in one explosion at Bogoslof, it was the sole indicator used to raise the alert level.
However, globally detectable volcanic lightning is not ubiquitous. According to Sonja Behnke, a scientist at Los Alamos National Laboratory who studies volcanic lightning, “It seems to be important to have external water to have the ice-charging mechanism.” She explains that Bogoslof has an external water source because its vent is submerged underwater. Similarly, Redoubt has a glacier at its summit, providing an external water source for ice-charging.
Van Eaton notes that another major challenge with volcanic lightning is understanding why some plumes have more than others. She points out, “Our sensor network [in Alaska] is still pretty sparse, so it’s only sensitive to major volcanic lightning events.” She continues, “This is why multi-pronged approaches are so important,” and adds that “no one method can stand alone.”
Further reading:
Behnke, S. A., Thomas, R. J., McNutt, S. R., Schneider, D. J., Krehbiel, P. R., Rison, W., and Edens, H. E. (2013). Observations of volcanic lightning during the 2009 eruption of Redoubt Volcano. Journal of Volcanology and Geothermal Research, 259, 214-234. https://doi.org/10.1016/j.jvolgeores.2011.12.010
Casadevall, T. J. (1994). The 1989-1990 eruption of Redoubt Volcano, Alaska: impacts on aircraft operations. Journal of Volcanology and Geothermal Research, 62, 301-316. https://doi.org/10.1016/0377-0273(94)90038-8
Schneider, D. J., Van Eaton, A. R., and Wallace, K. L. (2020). Satellite observations of the 2016-2017 eruption of Bogoslof volcano: aviation and ash fallout hazard implications from a water-rich eruption. Bulletin of Volcanology, 82, 29. https://doi.org/10.1007/s00445-020-1361-2
Van Eaton, A. R., Schneider, D. J., Smith, C. M., Haney, M. M., Lyons, J. J., Said, R., Fee, D., Holzworth, R. H., and Mastin, L. G. (2020). Did ice-charging generate volcanic lightning during the 2016-2017 eruption of Bogoslof volcano, Alaska? 82, 24. https://doi.org/10.1007/s00445-019-1350-5
- Living through the Loma Prieta earthquake - October 21, 2021
- The Great Quake Debate: an interview with seismologist and author Susan Hough - August 27, 2020
- Salton Sea Swarm quiets down - August 12, 2020