Tiegan Hobbs, Ph.D., Postdoctoral Seismic Risk Scientist, Temblor (@THobbsGeo)
Chris Rollins, Ph.D., Postdoctoral Researcher, Michigan State University
Citation: Hobbs, T.E. and Rollins, C., (2019), Earthquake early warning system challenged by the largest SoCal shock in 20 years, Temblor, http://doi.org/10.32858/temblor.035
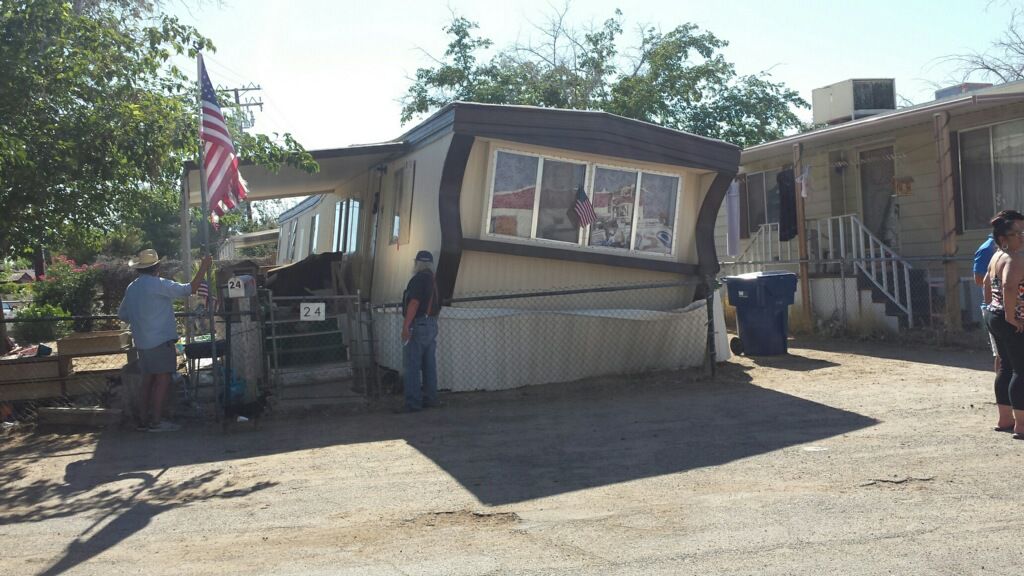
It was a long 4th of July for residents of Ridgecrest, California, as a M=6.4 earthquake struck just outside of town at 10:33 AM. While the earthquake fortunately appears to have caused no deaths, many homes and businesses in Ridgecrest were severely damaged, some beyond repair, like the home shown above. Shaking reverberated over a wide area and was felt as far away as Northern California, Phoenix and northern Mexico, according to the United States Geological Survey (USGS). In fact, so many people tuned in to the USGS website to report shaking or get information that the website was temporarily overloaded and went down.
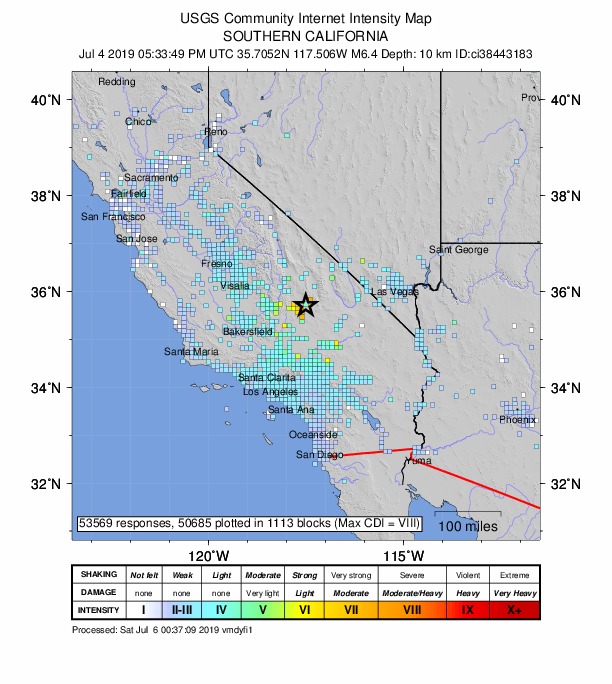
A nest of faults snaking through the High Desert
Rather than rupturing the well-known San Andreas fault, Thursday’s earthquake struck in the Eastern California Shear Zone, a flank of the plate boundary in California that accommodates about 30% of the relative motion between the Pacific and North American plates (the San Andreas picks up most of the other 70%). The Eastern California Shear Zone was the setting of the 1992 M=7.3 Landers and 1999 M=7.1 Hector Mine earthquakes, and Thursday’s tremor was a reminder that faults there compound the hazard posed by the San Andreas.
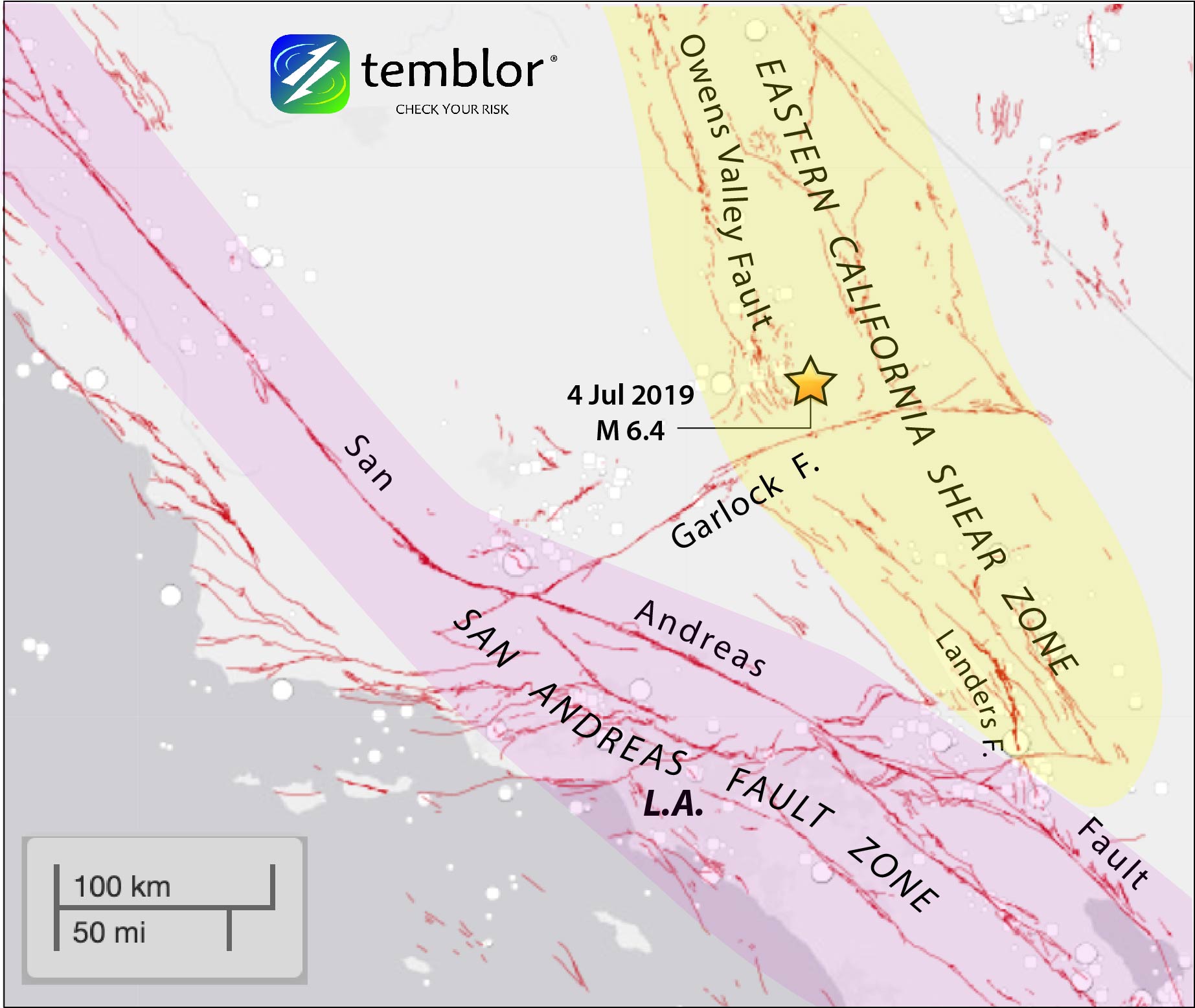
The July 4 earthquake appears to have ruptured two faults that intersect one another to form an ‘L’ shape, with one fault rupturing in a left-lateral sense (whichever side you are on, the other side moves to the left) and the other in a right-lateral sense (the opposite). These are called conjugate faults, and near-simultaneous rupture of two of them has been observed in California earthquakes before: in fact Thursday’s tremor was a near 180-degree-rotated version of a pair of M>6 earthquakes that struck near the Salton Sea in 1987, called the Elmore Ranch and Superstition Hills earthquakes [Hudnut et al., 1989]. One difference is that the 1987 quakes were separated by 12 hours, whereas it appears there was no pause between the two fault ruptures in Thursday’s quake.
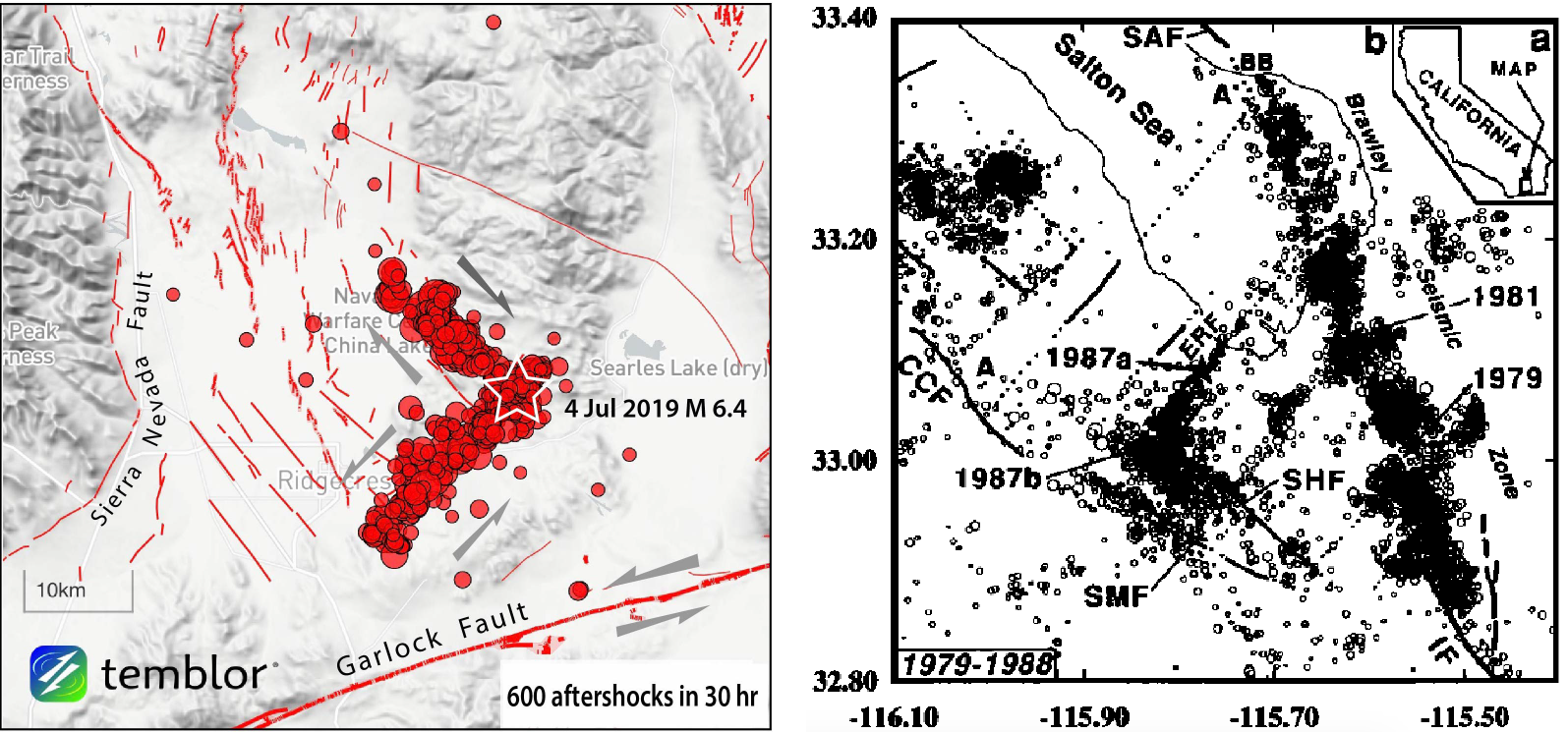
Why was no Earthquake Early Warning sent to Los Angeles residents?
ShakeAlertLA, an earthquake early warning app designed for residents of Los Angeles, was released to the public in January of this year. It interfaces with the USGS’s ShakeAlert information system, which detects and characterizes earthquakes. While ShakeAlert did correctly detect Thursday’s event, it estimated that shaking in Los Angeles would be weaker than the current cutoff intensity for issuing an alert to residents there, and so no warning was issued on the app. This left many wondering if the system was functional or well-suited to the city’s needs. In response, the City of Los Angeles pledged via Twitter to lower the threshold going forward.
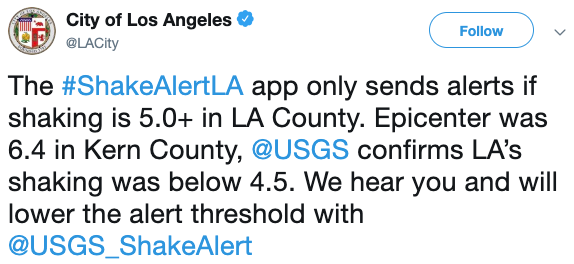
This change will presumably prevent this sort of confusion in LA in future earthquakes where the shaking is mild but noticeable, and ideally these will serve as opportunities for residents to practice drop, cover and hold on in real time and then think about what to do after the shaking subsides and how to prepare for a stronger earthquake in the future. An argument against lowering the alert threshold too much, however, is that users could become desensitized to alerts (think car alarms). The 4th of July earthquake in effect provides “a great [opportunity] to talk about prediction thresholds” and take stock of “what behavior [users] want,” according to Dr. Sarah Minson, geophysicist at the USGS.
Dr. Minson, who has led groundbreaking research quantifying the uses and limits of earthquake warning systems, likens the task to that of mandating hurricane evacuations, where the key is to “[make] sure you evacuated the people you really needed to get out.” Dr. Lucy Jones, head of the Lucy Jones Center for Science and Society, maintains that getting an alert that ‘you will soon feel a quake but it will not be damaging’ is nevertheless immensely valuable because it reduces anxiety. She considers the system effective as long as you receive an alert before you feel any perceptible shaking. Dr. Sarah MacBride, USGS social scientist, states that “advance warnings that shaking will be light are valuable only if that is the message conveyed in the alert.” What constitutes an actionable alert may also vary from user to user: a surgeon would want to know about any amount of shaking coming down the pipe, whereas a major data center can hardly afford to go down for any amount of time. These variabilities, however, do not obscure the importance of having a basic alert system that the public can work with.
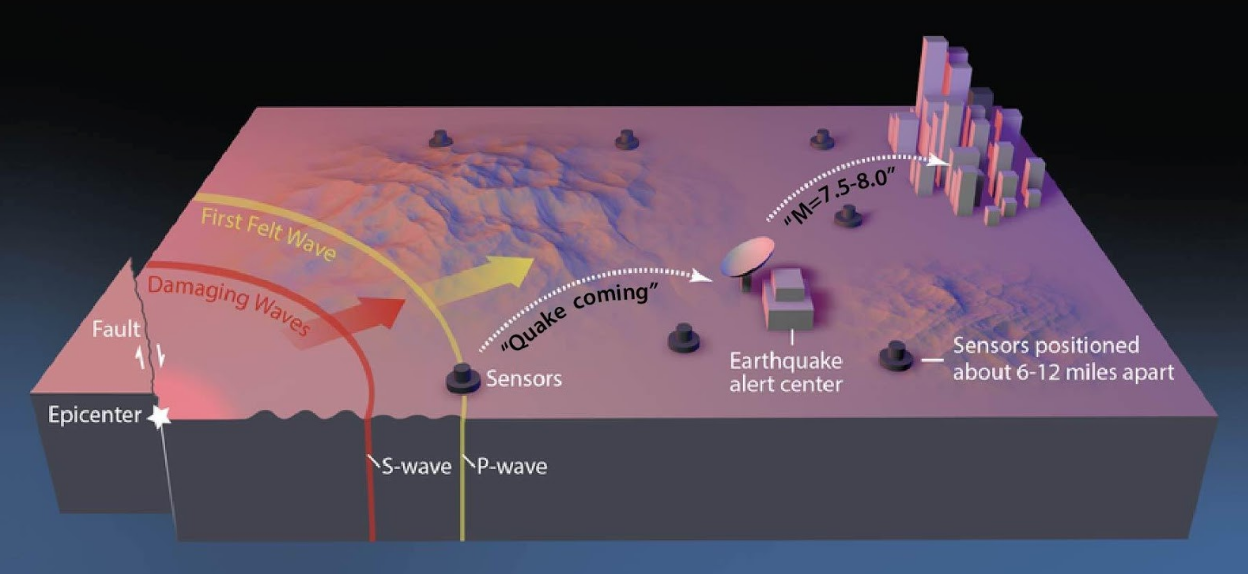
Dr. Robert De Groot, ShakeAlert coordinator for Education and Outreach, conveyed that “it is not yet clear whether any ShakeAlert beta users received an alert before moderate shaking arrived. We are investigating this now.” No warning would have been possible in Ridgecrest and the hardest-hit communities, unfortunately: they were so close to the epicenter as to be within what is known as the “blind zone,” in which the destructive waves arrive before a warning system has enough data to piece together that an earthquake is occurring (because that data comes from waves arriving at seismometers, which are only so densely distributed.) This is a well-known limitation of early warning, and so in fact the users that early warning can help the most are those who are outside the blind zone but at risk. This, importantly, includes all of greater Los Angeles for earthquakes on the San Andreas.
Misinformation and Earthquake “Predictions”
Just under 2 hours after the M=6.4 event, a tweet from Kern County Fire (@kerncountyfire) predicted another earthquake within the next 15 minutes. Despite strong calls from earthquake scientists and from the Incorporated Research Institutes for Seismology (IRIS) to take the tweet down, it has remained up, and has been defended by an official-looking twitter account called “CAL FIRE NEWS”. Of course, at one level the tweet was correct: There are certain to be felt aftershocks within two hours of a M=6.4 mainshock. But how useful is this warning?
Why the drama? With earthquakes, a prediction involves specifying the time, location, and size of an event, which is not currently possible in any practical sense. A forecast, by contrast, is an estimate of the likelihood of a particular event (read more about this from the Pacific Northwest Seismic Network). For example: the USGS forecasted that for 24 hours following 2:40 pm local time on July 4th, there is a 5% chance of an earthquake of magnitude greater than 6 occuring in the region of the Ridgecrest event. This forecast incorporated the data about the earthquake sequence up to that time as well as the best available knowledge about aftershocks, earthquake triggering, and California tectonics, and it provides a probability of an event that users can act on as they choose. Although first responders and scientists share the goal of lessening the impact of earthquakes, this event underscores the need for streamlined cooperation and communication between these groups prior to and during an emergency.
The Uniform California Earthquake Rupture Forecast (UCERF3) estimates that there is a 60% probability of a magnitude 6.7 earthquake in the Los Angeles area in the next 30 years, and a 72% chance in the San Francisco Bay Area. Such an event would be three times the size of the 4th of July quake. Protect yourself and understand your risk.
An M=4.0 foreshock 30 min before the mainshock
After the first of July, eleven earthquakes ranging in size from magnitude 0.5 to 4.0 were reported before the mainshock. Among these ‘preshocks,’ Prof. Haluk Eyidoğan (Istanbul Technical University) has found evidence of a M=4.0 earthquake that occurred 30 minutes before the M=6.4 quake, at the same location and depth, and with the same mechanism (sense of slip on the fault). This M=4.0 event may thus have constituted a foreshock.
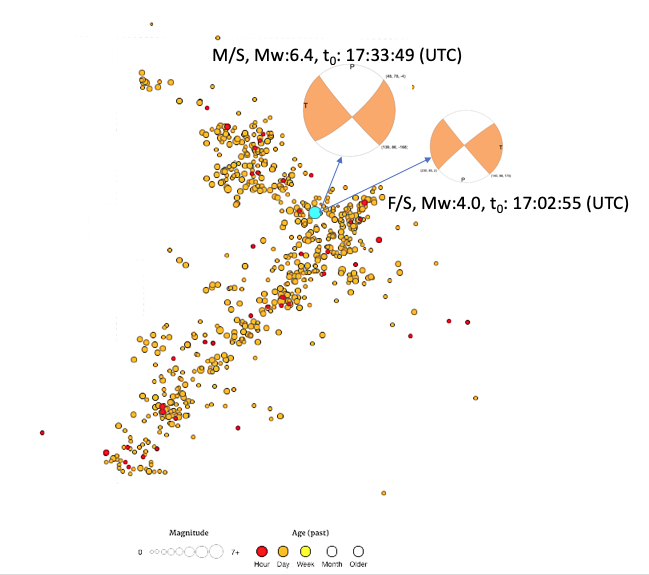
Unfortunately, as of currently, foreshocks are only identifiable after the occurrence of a mainshock: there is no way to know if an earthquake is going to precede a larger event before that larger event happens. Nevertheless, this M=4.0 event is sure to be closely studied.
Nudging the Garlock Fault Closer to Failure
The July 4th earthquake would have affected the state of stress on nearby faults, including the east-west trending Garlock Fault, a major fault running along the south side of the epicentral area. The distribution of aftershocks can be used to construct an approximate two-fault model of slip in the earthquake, which can be used to estimate these stress changes. We find that the earthquake likely increased the Coulomb stress on the central Garlock fault by close to 1 bar, sufficient for triggering [e.g., King et al., 1994; Ziv and Rubin, 2000].
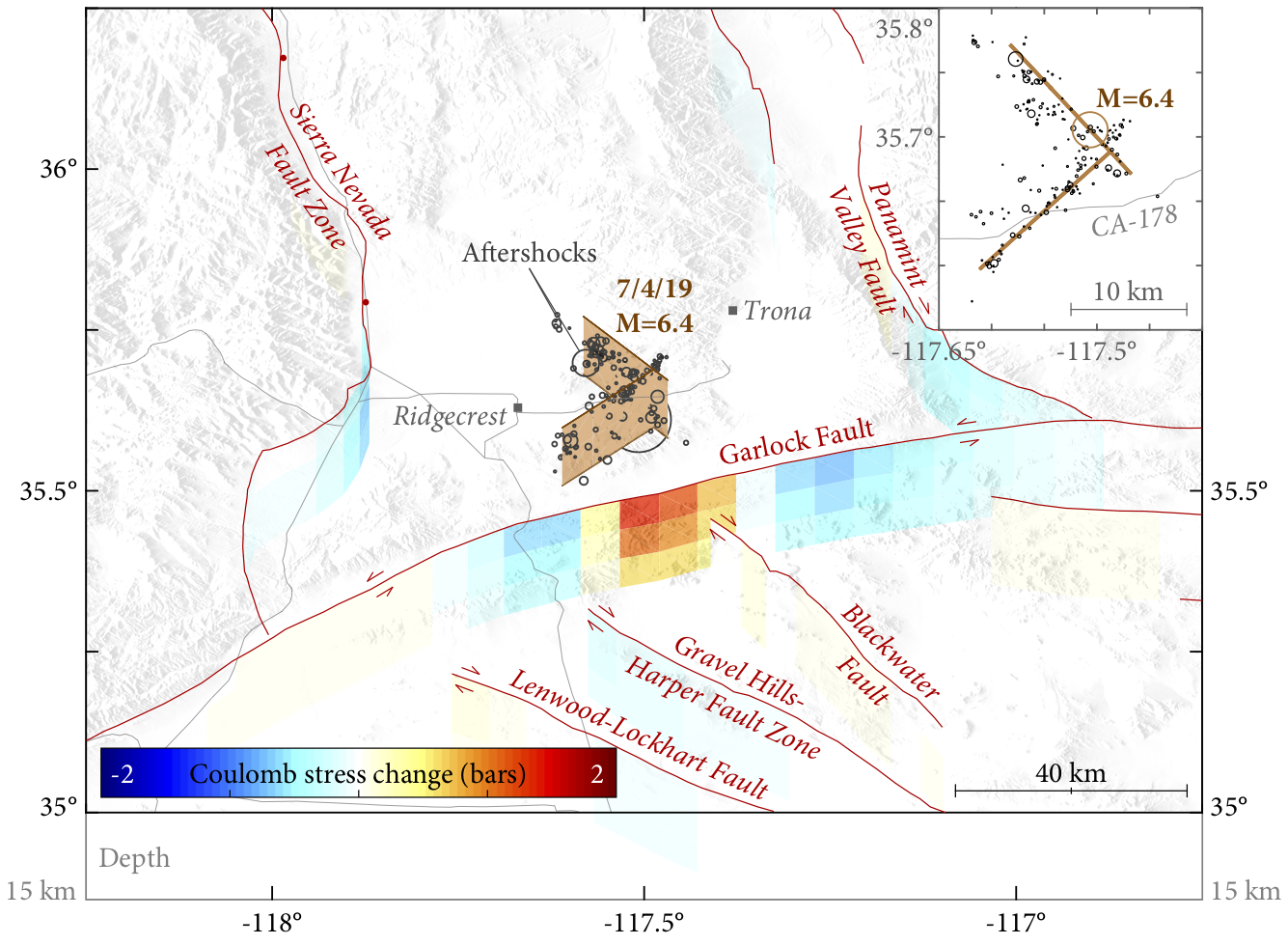
The Garlock Fault, in turn, extends southwestward to eventually intersect the San Andreas at the north end of the Grapevine, and it also extends further eastward from the epicentral area into the California Desert, spanning a total length of 280 km (170 mi). It effectively bisects the Eastern California Shear Zone (and nearly the state of California; see the third figure in this article) and is thought to serve as a mechanical link between the San Andreas and the faults in Eastern California [e.g., Dixon and Xie, 2018]. The last large shock on the central Garlock fault struck sometime between 1450 and 1640 AD [Dawson et al., 2003], and an end-to-end rupture on this fault would translate into a magnitude approaching 7.8. However, the Eastern California Shear Zone has warped and rotated the eastern Garlock (notice how far it is from near-perpendicular to anything around it, in comparison to the faults in Thursday’s quake) so that it might only be able to rupture if it were very slippery. So, whether it is ‘locked and loaded,’ or ‘dead and buried,’ is an open and now, gnawing, question.
And what if the Garlock were to rupture?
The immediate damage from a large earthquake on the central and western Garlock would depend on which way the fault ruptured. If the rupture propagated southwest from near Thursday’s event toward the Grapevine, it would likely send strong shaking into the Ventura and Los Angeles basins, which would amplify and prolong this shaking. If it propagated northeast from the Grapevine out into the desert, it would send shaking towards Las Vegas, which unfortunately also sits atop a sedimentary basin. Either way, the final static slip in such an earthquake (which is what we modeled above for the 4th of July quake) would torque the Mojave Desert in such a way as to promote failure on the San Andreas Fault near Palmdale, as shown below.
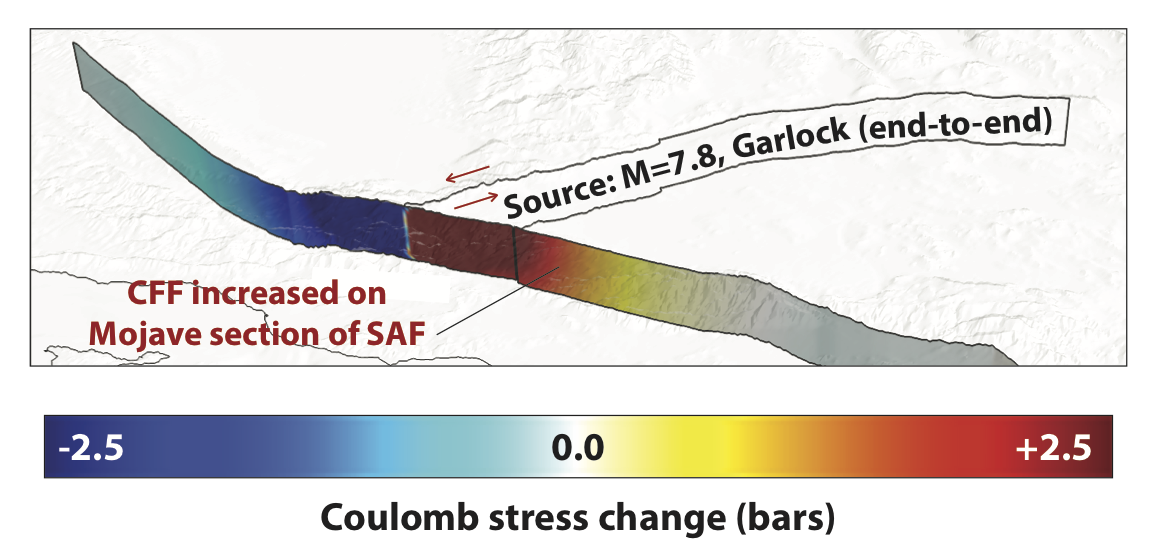
Earthquakes are often chain-reactions, whether in a decades-long domino-effect sequence (as on Turkey’s North Anatolian Fault), over 12 hours as in the Elmore Ranch/Superstition Hills case, or nearly immediately as in the Ridgecrest earthquake. The limitations of earthquake early warning, our inability to identify and detect foreshocks, and the inherent uncertainty about whether this shock will trigger a still larger one are all reminders that we can’t afford to take chances with earthquakes. Preparedness must start with each of us, through proper preparation, seismic retrofitting, and insurance.
References
Dawson, T. E., McGill, S. F., & Rockwell, T. K. (2003). Irregular recurrence of paleoearthquakes along the central Garlock fault near El Paso Peaks, California. Journal of Geophysical Research: Solid Earth, 108(B7), doi:10.1029/2001JB001744.
Dixon, T. H., & Xie, S. (2018). A kinematic model for the evolution of the Eastern California Shear Zone and Garlock Fault, Mojave Desert, California. Earth and Planetary Science Letters, 494, 60-68.
Hudnut, K. W., Seeber, L., & Pacheco, J. (1989). Cross-fault triggering in the November 1987 Superstition Hills earthquake sequence, Southern California. Geophysical Research Letters, 16(2), 199-202.
McAuliffe, L. J., Dolan, J. F., Kirby, E., Rollins, C., Haravitch, B., Alm, S., & Rittenour, T. M. (2013). Paleoseismology of the southern Panamint Valley fault: Implications for regional earthquake occurrence and seismic hazard in southern California. Journal of Geophysical Research: Solid Earth, 118, 5126-5146, doi:10.1029/jgrb.50359.
King, G. P., Stein, R. S., & Lin, J. (1994). Static Stress Changes and the Triggering of Earthquakes. Bulletin of the Seismological Society of America, 84(3), 935-953.
Ziv, A., & Rubin, A. M. (2000). Static stress transfer and earthquake triggering: No lower threshold in sight?. Journal of Geophysical Research: Solid Earth, 105(B6), 13631-13642.